WHERE IS THE CHEMOCLINE? - PART 1: INTRODUCTION
- Randy Blood
- Nov 23, 2020
- 3 min read
Anybody who has ever seen one of my talks knows that I enjoy discussing pyrite framboids. Indeed, since 2004, I have discussed framboids in organic-rich rocks some 20 times at various technical conferences, geological society meetings, and universities. In addition, my work on framboids appears in a handful of peer-reviewed journal articles, field trip guidebooks, and workshop texts. If you to learn more, much of this work can be found HERE.
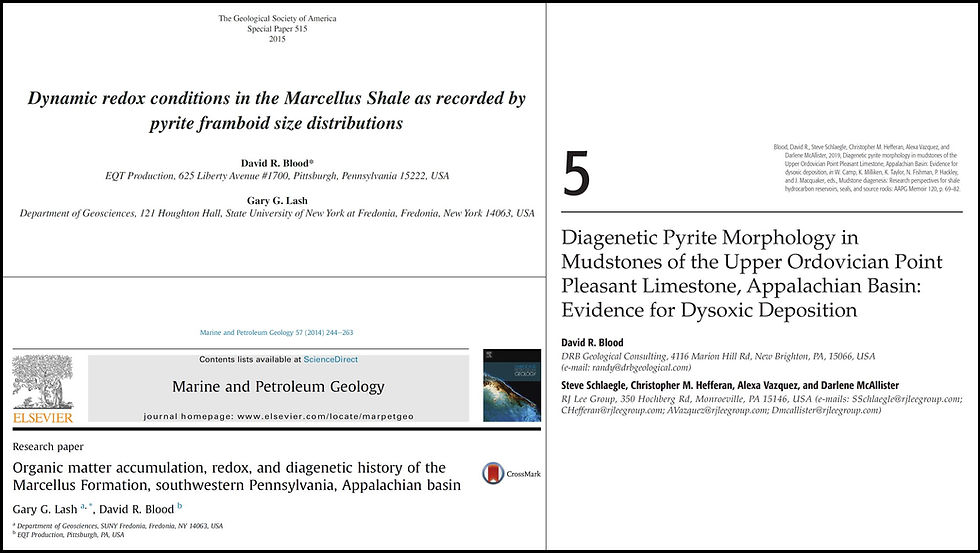
I’m interested in this topic because the size distribution of pyrite framboids is one measure by which we may determine the position of the chemocline, that is, the boundary separating oxygenated water from anoxic water in a basin. In general, a cohort of small (5-6 um), well sorted framboids suggests a chemocline that resides in the water column, while an assortment containing large and diverse framboids sizes suggests a chemocline residing at or near the sediment-water interface.
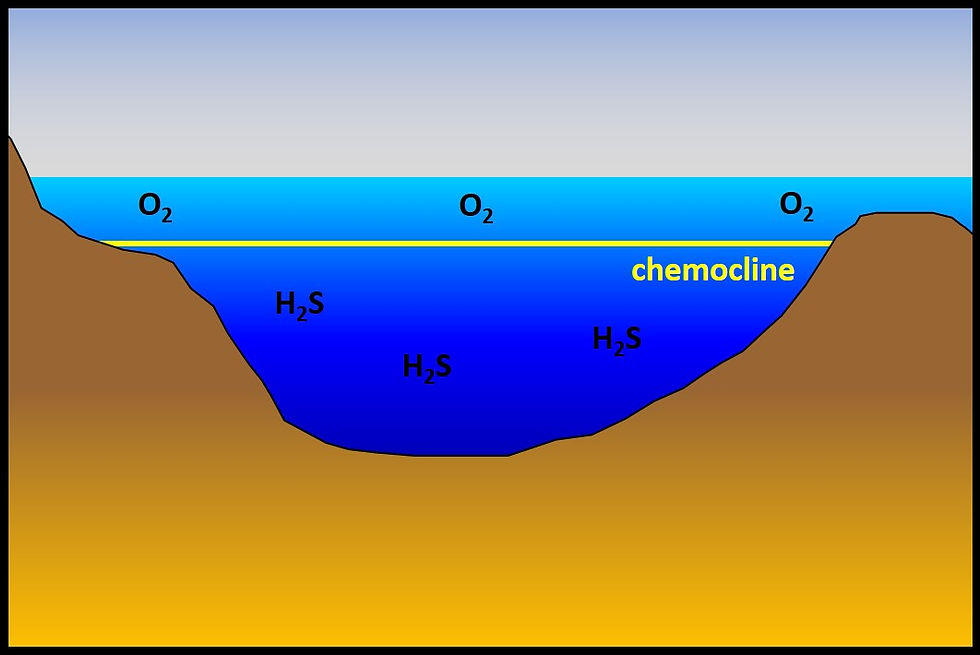
In the Marcellus Shale, the overwhelming majority of framboids are small, 5-6 um, but they do co-occur with a subordinate number of large framboids. The conclusion I have reached, is that these framboids represent deposition under conditions characterized by dominantly anoxic-euxinic (free hydrogen sulfide in the water) bottom waters. However, the assemblage of larger framboids suggests the position of chemocline fluctuated often and occasionally dropped to the sediment-water interface.
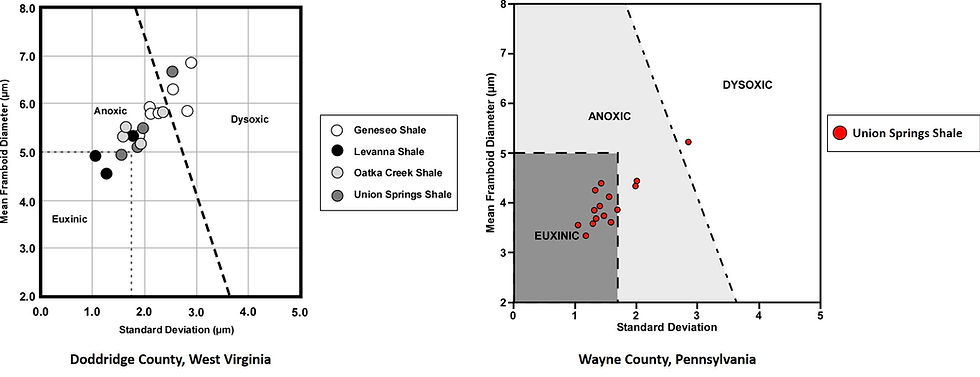
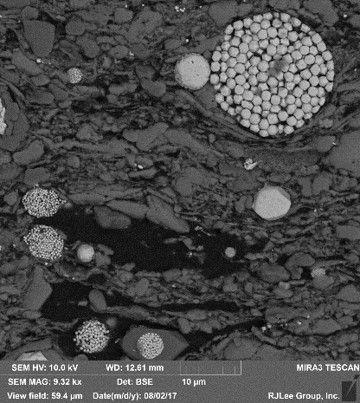
Over the course of presenting this research I have had numerous lively and thoughtful discussions about the implications of my research. Of all the questions I have been asked however, one in particular has always stuck with me: “If 5-6 um framboids indicate that the chemocline was in the water column, how do you know the chemocline wasn’t just 5-6 um above the sediment-water interface?” At its heart this is a difficult question to answer. It has been exceedingly difficult to understand water-depths in ancient basins (see Conant and Swanson, 1961, Baird and Brett, 1991, Kohl et al., 2014, and Smith et al., 2019 for examples) let alone the depths at which internal stratification occurs. However, the question has always been in the back of my mind when looking at these deposits both in the field and in core.
There is of course anecdotal information that gives us insight to the answer. One important point is that we often talk about fluid boundaries as though they are a sharp contact, when in actuality they should be more accurately described as a zone or layer. Indeed, in modern oceans the thermocline may have a thickness of 300 to 1,000 meters (Pond and Pickard (1983). Here are dissolved oxygen (DO) and hydrogen sulfide (H2S) profiles for the Black Sea (adapted and redrawn from Yemenicioglu et al., 2006) and the Upper and Lower Basins of the Pettaquamscutt River (adapted and redrawn from Wilken and Barnes, 1997):
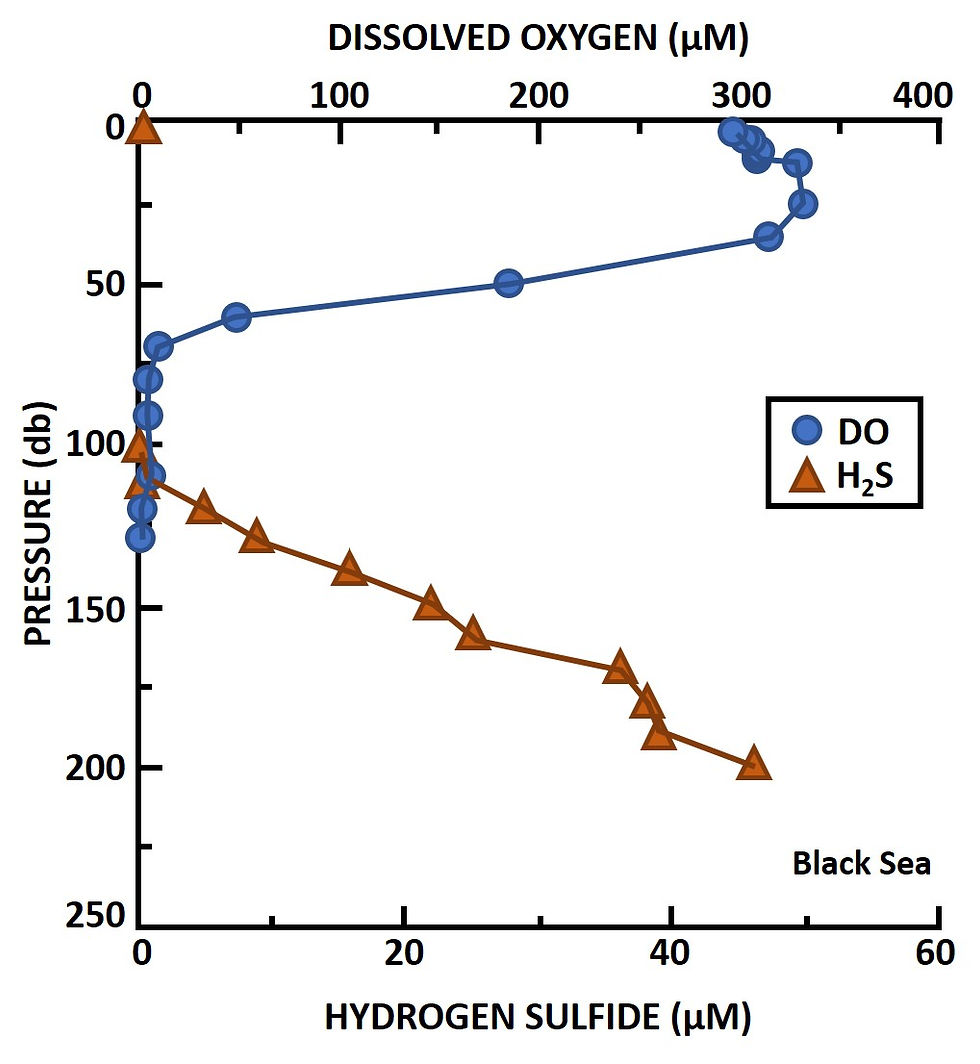
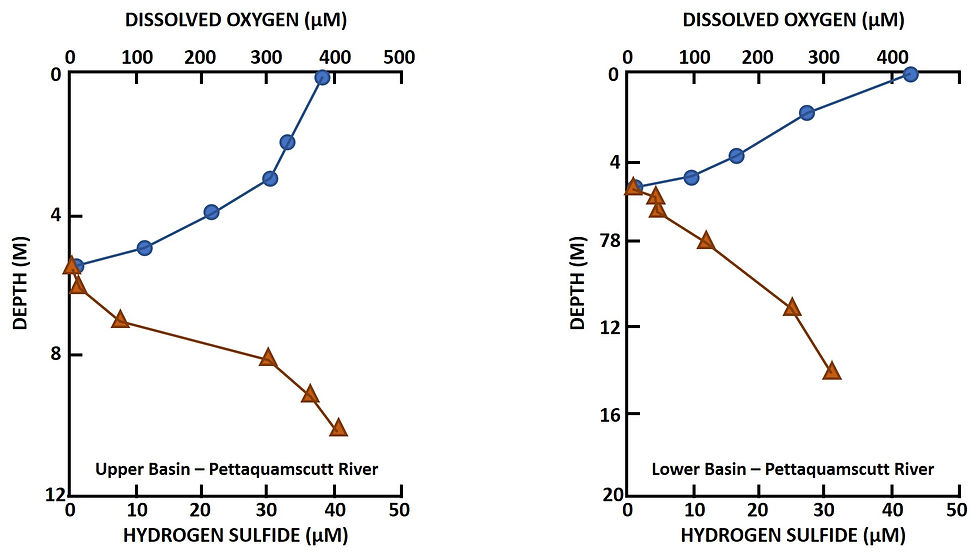
Moreover, it is important to note that a number of factors control the position of the chemocline, including basin connectivity to the global ocean, ventilation and the flux of oxygen to the deep basin, temperature, salinity, and the flux of hydrogen sulfide to the water column by bacterial sulfate reduction. All these factors suggest a chemocline boundary, sharp or not, prone to a fluctuating depth.
Finally, we also know that fossils of large predatory armored fish are commonly found in black shale deposits. Indeed, armored fish plates (see the example Dunkleosteus image from the Cleveland Museum of Natural History) have been found in the black shale facies of the Cleveland Shale and Huron Shale of Ohio, and the Dunkirk Shale, The Kellwasser Bed of the Hanover Shale, and black shales contained within the Gowanda Shale in New York. Such fossils point to a thriving ecosystem in the nektonic portion of the water column that overlies organic-rich muds mostly devoid of benthic fauna.
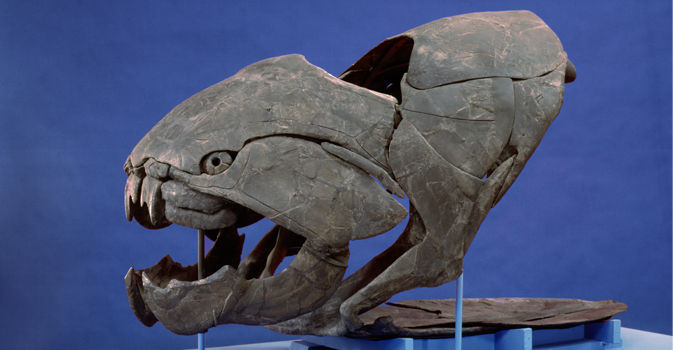
These observations, while insightful are not overly satisfactory for ascertaining where in the water column the chemocline occurs. While we still may not be able to achieve an exact answer, new research conducted by the author and others is beginning to provide further insight. In this five-part blog series I would like to address this issue in greater detail. In Part 2 we will review the framboid model, its pitfalls, and what the model suggests about the redox conditions of bottom-water in the Marcellus Shale. In Part 3 we will discuss pyritized fauna occurring in higher Hamilton Group strata, what it means for depositional environment, and its implications for water column oxygenation. In Part 4 we will discuss geochemical evidence that sheds light on the oxygen content of not only the bottom water, but the upper water column as well. Finally, in Part 5 we will summarize the data and my interpretations for what it all means, why we should care, and where we can go from here. With that, you have my sincerest thanks for reading, I hope you check out part 2, and remember to never stop exploring.
Comments